General design > 20 questions about fatigue
20 questions about fatigue
1. What is fatigue?
Fatigue is the weakening and eventual failure of a design structure due to repeated cycles of loading and unloading. To design for fatigue, it is important to consider the loading conditions, the design geometry, the manufacturing processes, and the fatigue properties of critical areas, such as welded joints.
2. How is fatigue load defined?
Fatigue load is defined by the stress range, number of stress cycles, the shape of the stress distribution, stress ratio, and the average stress. To define fatigue load, it is important to consider the time duration of the load and the minimum and maximum stress values. The stress range is the difference between the maximum stress and the minimum stress, and the stress amplitude is half of the stress range. The average stress is the mean value of the maximum stress and the minimum stress. The stress ratio, also called the R-value, is the minimum stress over the maximum stress. The shape of the stress distribution, which varies depending on loading conditions, is another important consideration in defining fatigue load.
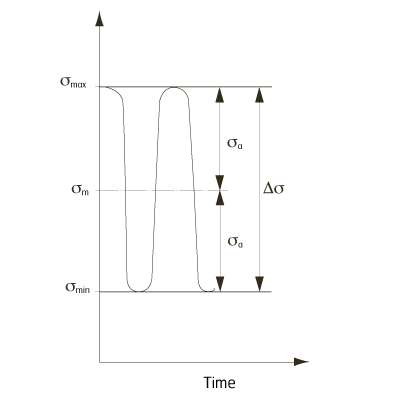
σmax = maximum stress level during a load cycle
σmin = minimum stress level
σm = mean stress level
σa = stress amplitude
Δσ = stress range
σmin = minimum stress level
R = σmin/ σmax stress ratio
R < alternating load
R ≥ pulsating load
3. What factors affect the fatigue strength of steel plate?
The fatigue strength of parent material is affected by various factors such as yield strength, surface roughness, the quality of critical edges, and notches. To ensure sufficient fatigue strength, it is important to consider the yield strength of the parent material and the surface roughness of the plate. The quality of critical edges and notches, such as holes for bolts or rivets, should also be carefully evaluated. SSAB Design Handbook provides guidance on how to select appropriate material in various applications under fatigue load.
4. What is steel toughness?
Toughness is the ability of materials to absorb energy and plastically deform before fracturing. Brittle materials can only absorb a low amount of energy and develop a small plastic deformation before fracturing. Ductile materials can absorb significantly more energy and withstand large plastic deformation before collapsing or fracturing. To ensure adequate toughness for a specific application, it is important to choose a steel with the appropriate minimum guaranteed toughness, depending on the expected operating temperature.
5. How is material toughness for steel evaluated?
Material toughness can be evaluated using different testing methods, including impact testing methods, drop weight testing, and fracture mechanics methods. Charpy V impact toughness testing is a commonly used method to measure the toughness of steel.
6. How can the fatigue design be improved?
Improving the fatigue design can involve adjusting the component geometry to achieve a more even load distribution and minimize stress concentrations. Additionally, surface finish and treatment can play a significant role in improving fatigue strength. Compressive residual surface stress, for example, can be induced through shot peening or cold working, while TIG-dressing can be used to improve the fatigue properties of weld joints. Choice of material is also an important consideration, with some materials exhibiting better fatigue properties than others, especially when there are no notches present.
7. Which methods can be used in dimensioning against fatigue?
Three common fatigue dimensioning methods are the Nominal Stress method, the Hot Spot method, and the Effective Notch method. These methods aim to calculate the stress levels in the component and compare them to the fatigue limit of the material. An alternative method is Design based on Fracture Mechanics, which considers the crack size and shape and the stress intensity factor to determine the component's fatigue life. Testing of materials, components and whole structures is also used as a complement to the dimensioning methods.
8. Why are smooth transitions important for better fatigue design?
Smooth transitions in geometry are important for better fatigue design because they reduce stress concentrations in components. Stress concentrations can cause local areas of high stress, which can result in fatigue cracking and failure at a lower number of cycles. Sharp transitions, such as corners or abrupt changes in geometry, can create stress raisers and increase the risk of fatigue failure.
9. How can fatigue be avoided in the design phase?
To avoid fatigue failure, it is important to analyze where cyclic loads result in high stresses in the structure and to avoid stress concentrations from sharp notches (such as welds) in those areas. The locations in the structure where cyclic loads result in high stresses can be identified through testing and stress measurements, traditional engineering methods (analysis and experience) or simulation.
10. What is the S-N curve?
The S-N curve is a graph of the fatigue behavior of a material which shows the relationship between the applied stress range and the number of cycles to failure. The stress amplitude is plotted against the logarithm of the number of cycles to failure for a given material. The S-N curve below shows an example of a S-N curve.
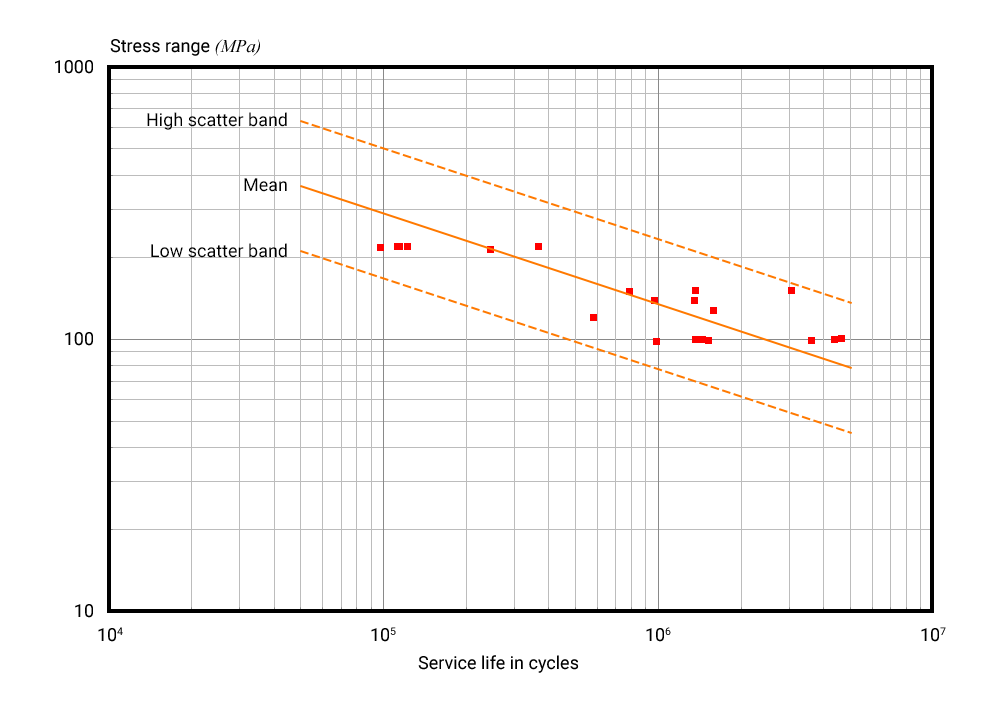
11. What is the difference between Constant Amplitude Loading and Variable Amplitude Loading?
Constant amplitude loading refers to a load that is constant over time in both amplitude and average stress, often represented as a sinewave. In contrast, variable amplitude loading is irregular over time, with varying amplitude and average stress.
12. How does strength of the parent material affect the fatigue strength of welded joints?
There is generally no impact on the fatigue resistance of welded joints from the parent material. The geometry of the weld alone determines the fatigue strength. However, at high stress levels, a weld in a high-strength steel can handle thousands of load cycles whereas a mild steel would collapse static. When designing welded joints, it is essential to consider various factors that affect the fatigue strength, including the geometry of the weld, loading conditions, stress concentration, and the presence of defects.
13. Why do welds have lower fatigue resistance compared to parent material?
Welds are geometric imperfections, like notches, and they can have defects, such as cold laps, undercuts and other surface-breaking defects. These defects cause stress concentrations, which can initiate fatigue crack growth, thereby causing the fatigue resistance of the welded joint to deteriorate. To mitigate the lower fatigue resistance of welds, one approach is to place them in low-stressed areas and so utilize the strength of the base material.
If this is not possible, consider changing the joint design or using another type of weld or connection. Improving welding procedures and quality control can also help to enhance the fatigue resistance of welds. It is crucial to perform a correct fatigue design analysis, often combined with testing, to assess the fatigue life of welded joints and ensure their safe and reliable performance in service.
14. How can the fatigue resistance of a weld joint be improved?
Welds are prone to stress concentrations and imperfections, which can lead to fatigue crack growth and subsequent failure. To improve the fatigue resistance of a weld joint, it is important to reduce these stress concentrations by minimizing the presence of geometric imperfections, such as sharp notches and defects like porosity and cold laps, and ensuring proper weld fusion and penetration.
One way to enhance the fatigue resistance of a weld joint is to enhance the weld quality by improving the geometry of the weld seam or by adjusting the welding parameters, such as the welding speed and current, to achieve better fusion and penetration. Another way is to apply post-weld treatment methods, such as TIG-dressing, shot peening or grinding to reduce the surface roughness and induce compressive stresses that can retard fatigue crack initiation and crack growth.
15. How does weld quality affect fatigue strength?
The quality of the weld, particularly at the weld toe or root, has a significant impact on the fatigue strength of a welded joint. This is because these areas are highly stressed, and any defects or stress concentrations can act as sites for fatigue crack initiation and propagation. In contrast, the center of the weld seam is usually less critical in terms of fatigue strength.
16. What is the fatigue strength of cut and sheared edges?
The fatigue strength of cut and sheared edges is directly influenced by the quality of the cut and the tools used to make it. When materials are cut or sheared, surface imperfections and microcracks may be left on the edges, which can lead to a reduction in fatigue strength. To improve the fatigue strength of cut and sheared edges, it is important to ensure quality assurance and maintenance of the cutting or shearing tools. This can minimize surface imperfections and microcracks that can compromise the fatigue strength of the edges. Additionally, post-processing techniques such as grinding or polishing may be used to further reduce surface roughness and improve fatigue strength.
17. How does surface quality affect the fatigue strength of parent material?
The surface quality of parent material can significantly affect its fatigue strength. A smoother surface can increase fatigue resistance, whereas rough surfaces with less surface finish and with defects like scratches, cracks, or porosity can act as stress concentration points and reduce fatigue strength.
18. How can corrosion affect fatigue resistance?
Corrosion can have a significant impact on the fatigue resistance of steel. Fatigue cracks typically initiate from the surface, and corrosion can produce a rougher surface structure, reducing fatigue resistance. Additionally, corrosion pits or cracks can accelerate the crack growth rate, further reducing fatigue resistance.
19. What is Post Weld Treatment (PWT)?
Post Weld Treatments (PWT) are a range of techniques used to improve the fatigue strength of welded joints. These methods often focus on improving the weld toe geometry, reducing stress concentrations, and/or creating protective compressive residual stresses in the area surrounding the weld. Examples of PWT methods include TIG dressing, HFMI (High Frequency Mechanical Impact), burr grinding, peening, and shot blasting.
20. How can Post Weld Treatment (PWT) be used for better fatigue resistance?
Post Weld Treatments (PWT) are applied to welded joints to enhance their fatigue strength. PWT methods aim to reduce stress concentration and/or introduce compressive residual stresses in the area surrounding the weld. The methods should only be applied to critical areas. Common PWT methods include TIG dressing, HFMI (High-Frequency Mechanical Impact), burr grinding, peening, and shot blasting. These methods are typically applied locally, focusing on areas where higher fatigue strength is needed, such as the weld toe or areas with high stress concentration. Proper application of PWT methods is crucial to achieve the required results, and guidelines and best practices should be followed to ensure good quality. To improve the fatigue resistance of welded joints, it is important to choose the appropriate PWT method based on the material being welded, the welding process used, and the required outcome. TIG dressing is often used to smooth out the weld toe, while methods like HFMI and peening can further introduce compressive residual stresses to improve fatigue strength.
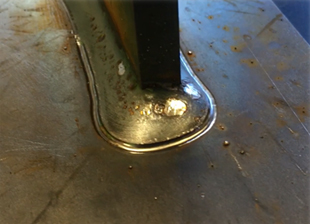
The information in this report is only applicable to SSAB’s products and should not be applied to any other products than original SSAB products.
This report provides general results and recommendations for SSAB steel products. This report is subject to SSAB’s Terms of Use. It shall be the user's responsibility to verify that the information contained herein is correct and is suitable to be used for the particular purpose and application of the user. The report is intended to be used by professional users only who possess adequate expertise, qualification and knowledge for the safe and correct use of the results and recommendations in this report. This report is provided “as is”. The use of the report is at user’s own discretion and risk and that users will be solely responsible for any use of this report. SSAB disclaims any liability for the content or potential errors of this report, including but not limited to warranties and condition of merchantability or fitness for a particular purpose or suitability for individual applications. SSAB shall not be liable for any kind of direct or indirect damages and/or costs related to or arising therefrom, whether special, incidental, consequential or directly or indirectly related to the use of, or the inability to use, the report or the content, information or results included therein.