General design > 20 questions about structural load resistance
20 questions about structural load resistance
1. How much stronger will my upgraded structure be?
The strength of the upgraded structure depends mainly on the yield strength of the material in it, how the geometrical features of the structure are changed, and on how the loads are introduced into the new structure. If the geometry of the upgraded structure remains unchanged and instability or fatigue are not an issue, the strength of the structure will increase proportionally with the increase in yield strength of the material. However, if the aim of the upgrade is to reduce weight by decreasing the thickness of the material, instability and fatigue become more likely and must be carefully considered. In such cases, the strength of the upgraded structure may not increase proportionally with the increase in yield strength, and the effects of instability and fatigue must be considered.
2. How much can the weight be lowered by using high-strength steels?
The weight reduction that can be achieved by using high-strength steels in a structure depends on various factors. These include the geometry of the structure and the yield strength of the high-strength material used in the upgrade. The potential weight reduction can be estimated using the rule of thumb, which is based on the square root of the yield strength ratio between the high-strength steel and the mild steel. However, it is important to note that factors such as the maximum allowed deflection in the structure, reduced fatigue life, and various instability phenomena can limit the potential weight reduction.
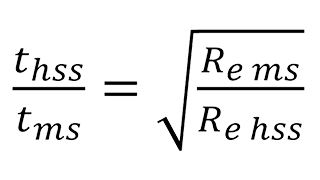
where:
thss = thickness of the high-strength steel
tms = thickness of the mild steel
Re ms = yield strength of the mild steel
Re hss = yield strength of the high-strength steel
3. Is it possible to have a lighter and stronger structure at the same time?
Yes, it is possible to achieve a structure that is both lighter and stronger by using higher strength steel grades. The higher strength of the material can lead to a reduction in weight while also increasing the load-carrying capacity of the structure. However, it is important to note that achieving a lighter and stronger structure also depends on other factors, such as the applied loads, the geometry of the structure and the thickness of the material.
While using higher strength materials can result in a lighter and stronger structure, it is essential to address potential challenges, such as fatigue, deflection, and instability, which may arise from this approach.
4. What is bending moment?
Bending moment is the reaction induced in a structure when an external force or moment is applied, causing the structure to bend. The force or moment creates a bending stress within the structure, which deforms it.
5. What is bending moment resistance?
Bending moment resistance is the maximum bending moment that a structure can withstand before it fails or reaches its limit state. It is an important parameter in the design of load-bearing members, as it determines the maximum load that a structure can support without failure. The bending moment capacity of a structure depends on various factors such as the material properties, geometry, load application, and boundary conditions.
6. How can the bending moment capacity of a beam be increased?
The bending moment capacity of a beam can be increased by using higher strength steel grades and changing the beam's cross-section geometry. The section modulus, a geometric property of the cross-section, measures the beam's resistance to bending. It can be increased by raising the height or width of the beam, or by using thicker materials. However, factors such as yielding, local buckling, and lateral torsional buckling can also affect the bending capacity. Therefore, careful consideration of these factors is necessary to ensure that the beam can withstand the maximum loads.
7. Why does an I-beam look like an I-beam?
The name "I-beam" comes from the similarity between the shape of the cross section of an I-beam and the shape of the letter “I” in the alphabet. This shape allows thicker thicknesses in the top and bottom plates than in the middle. It is usually the optimal way of using material in a beam subjected to bending loads. This means I-beams allow for a high strength-to-weight ratio, making them an efficient choice for load-bearing structures.
The shape of an I-beam provides several benefits. The thicker top and bottom plates, known as flanges, are further apart from each other than the middle section, called the web. This means the material is mostly placed where the bending stresses are high. This design results in the flanges being able to resist more bending stress than the web, while still maintaining a relatively low weight. This distribution of material makes I-beams ideal for carrying heavy loads over long spans.
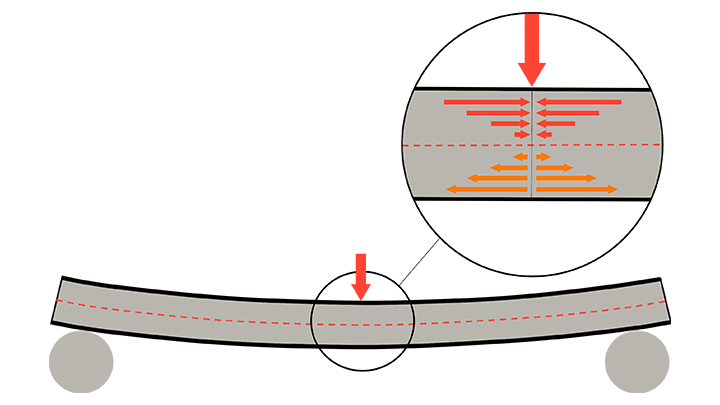
Close to upper and lower flanges are high stress areas, where most of the material is positioned.
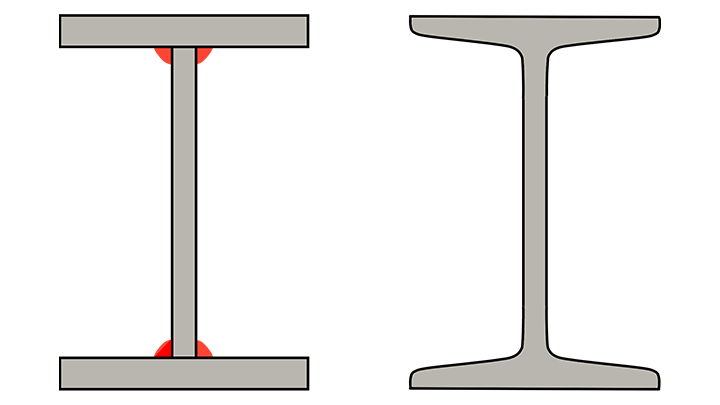
Welded I-profile vs. hot-rolled I-profile.
8. Which cross-sectional shape has the highest bending moment capacity for a beam?
The I-beam's design allows for different thicknesses in its flanges compared to its web, which leads to dedication of most of the section area to the most critical area of the beam. The function of the single web is to keep the flanges apart, while the flanges provide enough cross sectional area far from the neutral line of the I-beam. This assembly allows for greater bending moment capacity than other cross-sectional shapes with the same weight, making it a popular choice for structural applications.
9. How does instability affect load resistance?
For components subjected to compression, instability can cause component failure at a stress level lower than the nominal yield strength of the material. It is common to differentiate between local instability, such as local buckling or shear buckling, and global instability, such as flexural buckling, lateral torsional buckling or flexural torsional buckling. The risk of local or global instability occurring depends on factors like the width-to-thickness ratio of the cross-section, the length-to-stiffness ratio of the component, initial bow imperfections, the presence of residual stresses, etc. Since both local and global instability can be decisive for the load resistance of a component in compression it needs to be considered when designing such components.
10. Is sufficient bending moment capacity enough for a structure to withstand the applied loads?
No, it is not. A structure can be subjected to various types of loading, including shear, torsion, and fatigue, which require the structure to have the capacity to resist these forces. Additionally, the deformations induced by the loads should not exceed the allowable limits for the structure to perform safely. This makes it important when designing a structure to consider all types of loading and ensure that the structure can withstand them.
11. What are loads in structural engineering?
In structural engineering, the term "loads" usually refers to the external forces applied to a structure. These forces can be classified as dead loads (permanent loads such as the weight of the structure itself), live loads (variable loads such as the weight of transported goods, people or equipment), and environmental loads (loads such as wind or earthquake). The unit used to measure the amount of force is Newton (N), and the direction of applied forces is typically defined using global axes. The load can also be applied as a bending moment or torque expressed by the unit Newtonmeter (Nm).
Engineers use a variety of tools and techniques to capture the loads and handle them during the design phase. First, it is essential to accurately determine the magnitude, direction and duration of all potential loads that the structure may be subjected to. This can be done by conducting a thorough analysis of the environmental conditions, expected usage and other relevant factors that could affect the structure.
Once the loads have been identified, engineers can then use various tools, such as classic analytics, finite element analysis (FEA) or computational fluid dynamics (CFD), as well as testing and feedback from usage, to simulate the behavior of the structure under different loading scenarios. This can help to identify potential failure modes and guide the design of the structure to ensure that it can withstand the expected loads without exceeding its maximum stress limits.
During the design phase, it is also important to consider the dynamic effects of the loads, such as the impact of live loads or the resonance induced by environmental loads, such as wind. These effects can lead to increased stress and deformation in the structure and must be accounted for to ensure structure safety.
Finally, engineers can use various design measures to handle the loads, such as selecting appropriate materials, increasing the size or number of structural members, or incorporating support structures, such as trusses or braces, to help distribute the loads. By taking a comprehensive approach to capturing and handling loads during the design phase, engineers can ensure that the structure will perform safely and effectively under the expected conditions.
12. What is the difference between load resistance and load capacity?
Load resistance refers to the ability of a structure to withstand external loads without failing. It is a structural property that follows the rules of physics and is determined by the material strength and dimensions of the structure. On the other hand, load capacity is the maximum load that a structure is designed or allowed to carry, which is often determined by regulations or manufacturers.
Load capacity can be affected by factors such as safety factors, environmental conditions, and other design considerations. While load resistance is a fundamental property of the structure, load capacity is more of a regulated or contractual limit and can vary from one country and industry to another. By considering both load resistance and load capacity during the design phase, engineers can ensure that the structure is safe and can perform its intended function.
13. What is standard load?
In some contexts, regulations and standards define the minimum load a structure should be able to withstand before failure. This requirement is often referred to as the "standard load." For example, the rear bumper of a trailer must be designed to withstand a standard load as specified by the relevant regulations.
Standard loads vary by product and are typically specified in industry-specific regulations and standards. In the United States, the American Society of Mechanical Engineers (ASME) and the American National Standards Institute (ANSI) are two organizations that publish standards for various products. The European Union has similar standards through organizations such as the European Committee for Standardization (CEN) and the European Committee for Electrotechnical Standardization (CENELEC). In Asia-Pacific (APAC), the International Organization for Standardization (ISO) and the International Electrotechnical Commission (IEC) are two prominent organizations that develop standards. Manufacturers, designers and engineers can refer to these organizations and their publications to ensure that their products meet the minimum load requirements specified for their respective industries.
14. What are real loads vs. standard loads?
Standard loads are defined by regulations and standards, and they represent the loads that a structure must be able to withstand to comply with those regulations and standards. For example, the loads that a bridge should withstand to meet safety standards are defined in the relevant regulations. Accordingly, engineers design the bridge to withstand those standard loads.
In contrast, real loads are the actual loads that a structure experiences in the real world. These loads may differ from the standard loads if any standard is applicable. For instance, a truck frame is subject to fatigue loads in the real world, which may be not covered by the loads specified in a standard or by earlier design work. It is important to ensure that the structure can withstand the actual loads that it is likely to encounter in its intended use. Therefore, evaluating the real loads through field tests and the corresponding performance of the structure through simulations and practical tests is necessary to ensure that it is safe and meets the necessary requirements throughout its lifespan.
15. What are constant loads vs. variable loads?
In structural engineering, loads can be classified into two categories: constant loads and variable loads. A constant load, also known as a static load, is a load that remains constant and does not change over time. This type of load can be calculated and designed for accurately. A variable load, on the other hand, is a load that changes over time and can be unpredictable. These loads can include environmental factors, such as wind and earthquakes, or changes in the usage of a structure over time.
For example, the applied load (force) on the chassis of a parked trailer equals the weight of the burden and is a constant load. If this trailer runs on a road, the applied load on the chassis oscillates around the weight of burden due to dynamic effects (vibration, road condition, bumps, etc.). The oscillations or variation in an applied load depends on the severity of dynamic effects.
16. What is load resistance vs. dent resistance?
Load resistance refers to the ability of a structure to resist external forces that could cause global failure, such as plastic deformation, buckling, or complete collapse. Dent resistance, on the other hand, is the ability of a material to resist damage from concentrated impact loads that cause indentations or dents.
Dent resistance is essential in industries such as automotive and aerospace, where materials must withstand concentrated impact loads while maintaining their structural integrity. Engineers can test dent resistance using various methods, including dropping a weighted ball onto a material sample and measuring the resulting deformation. The results of these tests can help optimize material selection and design in these industries.
17. What is load resistance vs. corrosion resistance?
Load resistance mostly refers to the resistance of a structure against external forces which cause global failure of the structure such as plastic deformation, buckling, or complete collapse. Corrosion resistance refers to the resistance of the material to interaction with a chemical environment in a way that negatively affects the material property needed for the proper functionality of the structure.
Corrosion can reduce the actual load resistance due to reduced thickness or rougher surface.
18. How is the safety factor correlated to the yield strength?
Structures are often designed with a safety factor to ensure that they can withstand external loads or environmental factors without failure. This safety factor is typically expressed as a ratio between the maximum load a structure can bear and the expected load it will experience during use. One way to achieve this safety factor is by selecting a material with a yield strength higher than the minimum required level of strength.
Yield strength is an important property of a material that determines its ability to withstand stress and deformation without permanent damage. The guaranteed value of the yield strength is typically provided by the manufacturer and is based on standardized testing methods. Engineers can use these values to determine the appropriate material for a given application and ensure that the desired safety factor is achieved. However, it is important to note that other factors such as the required safety margin against failure due to the loads, manufacturing defects, material degradation, and unexpected loads should also be considered in the design process.
19. How can the load resistance of a structure be calculated?
Structural analysis is a branch of engineering which focuses on the performance of structures, including load resistance. There are manual calculation or computer simulation methods which allow engineers to evaluate the load resistance of a structure.
Structures must be able to withstand various loads, such as weight, wind, and environmental factors, without collapsing or deforming. To determine the load resistance of a structure, engineers use structural analysis techniques, which can be either analytical solutions (traditionally manual calculations) or numerical computer simulations (finite element analysis).
Analytical solutions, also known as classic engineering methods, are traditionally used to calculate the load resistance of structures. These methods are based on mechanics and the theory of strength, and empirical and test results. Mathematical equations are used to calculate, for example, the stresses and strains within a structure under various loads.
However, computer simulations, such as finite element analysis (FEA), are also used, using more complex simulation models. FEA is a numerical method that divides a structure into small elements, which are then analyzed for stresses and strains. This allows engineers to evaluate the load resistance of a structure under different loading conditions and simulate the design changes made to ensure its safety. FEA is a widely-used tool in engineering design due to its efficiency when analyzing complex structures, however, expertise is often needed to validate the models and to interpret the results.
20. Is the Finite Element Method (FEM) a reliable method for evaluating load resistance?
The Finite Element Method (FEM) is widely used to simulate and analyze the performance of complex structures under different loading conditions. It is generally considered a reliable method for evaluating load resistance. However, the accuracy of FEM analysis is influenced by several factors, such as the accuracy of input data, the appropriate selection of analytical simulation technique, and the level of detail and accuracy of components modeled.
To ensure the reliability and accuracy of FEM results, it is important to carefully validate and verify them. This can be done by comparing the FEM results with experimental or field data, or by using other analytical methods. By doing so, engineers can identify any discrepancies and adjust the simulation model accordingly to improve its accuracy. Overall, FEM remains a powerful tool for evaluating load resistance in complex structures, provided that appropriate care is taken in the selection of input data, the simulation technique and the validation process.
The information in this report is only applicable to SSAB’s products and should not be applied to any other products than original SSAB products.
This report provides general results and recommendations for SSAB steel products. This report is subject to SSAB’s Terms of Use. It shall be the user's responsibility to verify that the information contained herein is correct and is suitable to be used for the particular purpose and application of the user. The report is intended to be used by professional users only who possess adequate expertise, qualification and knowledge for the safe and correct use of the results and recommendations in this report. This report is provided “as is”. The use of the report is at user’s own discretion and risk and that users will be solely responsible for any use of this report. SSAB disclaims any liability for the content or potential errors of this report, including but not limited to warranties and condition of merchantability or fitness for a particular purpose or suitability for individual applications. SSAB shall not be liable for any kind of direct or indirect damages and/or costs related to or arising therefrom, whether special, incidental, consequential or directly or indirectly related to the use of, or the inability to use, the report or the content, information or results included therein.